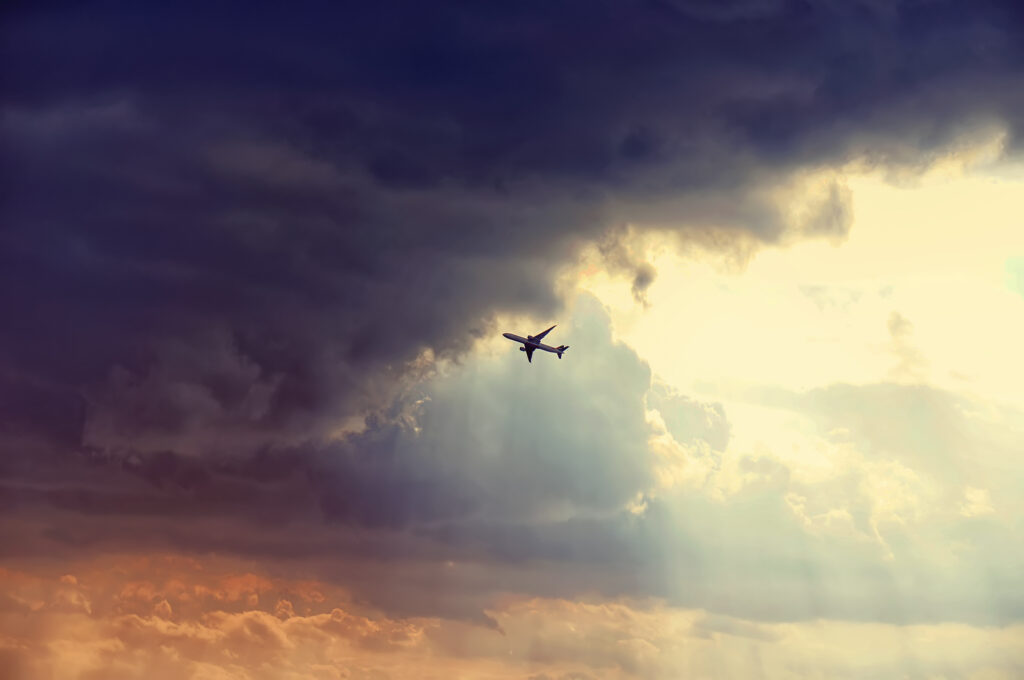
A series of thunderstorms and windshear-related air carrier accidents culminating with the crash of Delta 191 during final approach into DFW had a profound impact of aviation safety globally. These aircraft accidents motivated a partnership between the Federal Aviation Administration (FAA) and National Aeronautics and Space Administration (NASA) to investigate microbursts and provide pilots with adequate warning. The research, technological development, instillation and regulatory requirements, and pilot training associated with airborne windshear and alert was spearheaded by the Airborne Windshear Detection and Avoidance Program (AWDAP). Today, airborne weather radar, and windshear warning/flight guidance system and detection and avoidance system are required equipment for large-transport category aircraft used for air-carrier operations (14 CFR § 121.357 & 121.358).
The F-Factor
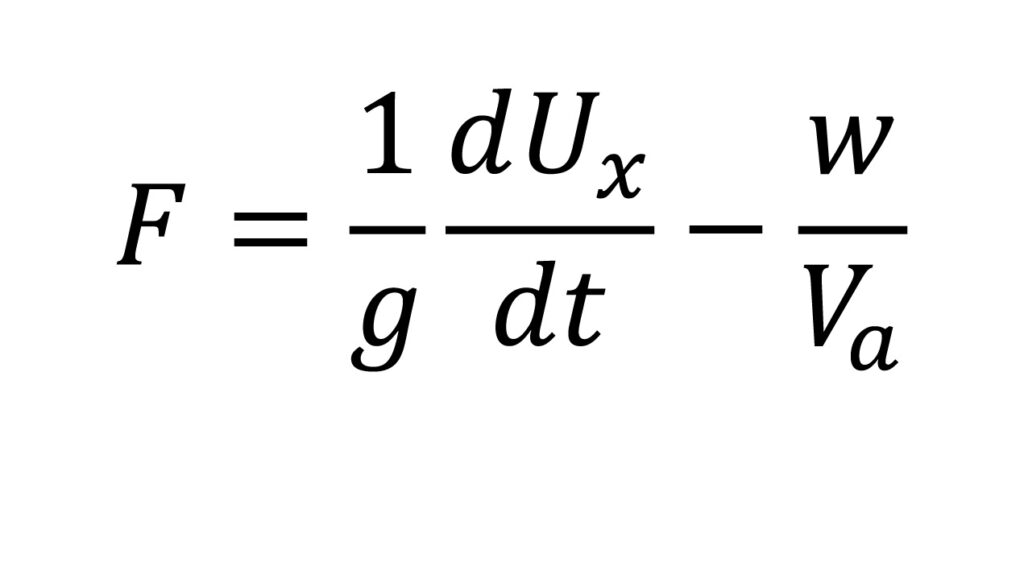
The F-Factor was developed by Bowles (1990). F represents the actual or predicted instantaneous loss of rate-of-climb performance, g the gravitational acceleration, Ux the headwind along the aircraft’s longitudinal glide-path, w the vertical wind velocity, and Va the aircraft speed. A positive F value indicates a loss of climb performance attributable to a combination of horizontal windshear that reduces the magnitude of the relative windspeed over the airfoil and thus reducing life, or downdrafts that force the aircraft to the ground. An F-Factor ranging between 0.1-0.15 or more that approximately corresponds to over a 1000-1500 ft/min loss of climb performance for a typically large transport category aircrafts are used to provide windshear advisories.
The Equipment
Airborne windshear warnings and advisories are generated either by reactive windshear (RWS) and/or predictive windshear detection (PWS). As the name suggests, reactive windshear alerts the flight crew that the aircraft is encountering windshear and cannot predict windshear that may be ahead of the aircraft. Predictive windshear utilizes the airborne weather radar to scan ahead of the aircraft. If the doppler radar identifies patterns conducive for windshear, the flight crew is alerted before the aircraft enters these dangerous windshear conditions.
Reactive windshear generates alerts and warnings by comparing the aircraft’s inertial and aerodynamic data. The objective is to evaluate changes in the aircraft’s total energy and alert the pilots when pre-determined performance-loss thresholds are exceeded. The aircraft system and equipment used to generate windshear advisories depends on the type aircraft and equipment. For instance, the Bombardier Global 6000 (BBD-700) used the terrain awareness and warning system (TAWS) while most Airbus aircraft types rely on the Flight Augmentation Computer (FAC) for reactive windshear and escape guidance. These systems typically process attitude, airspeed, accelerations, angle-of-attack (AOA), glideslope, and configuration for reactive windshear advisories.
Predictive windshear depends on doppler Radio Detection and Ranging (RADAR). Radar is an active remote sensing technique that measures the duration and intensity of the returning echo signal. The duration determines distance while the corresponding intensity indicates the presence of moisture, i.e., areas with active weather. The weather radar provides the velocity of the moisture particle by measuring the doppler shift i.e., how quickly a moisture particle is moving either toward or away from the radar receiver. Airborne weather radar manufactures implement the -Factor that is required for regulatory approval as per the FAA Technical Standard Order (TSO-C63d). Once the manufacturer has demonstrated regulatory compliance, the equipment is approved by the regulatory authority such as the FAA in the United States.
Windshear Avoidance
From a meteorological perspective, windshear (horizontal and/or vertical) is prone to exits in convective environments such as thunderstorms and microbursts, frontal activity, jet steams (including nocturnal- and the mid-latitude cyclone induced-low level jet), and mountain waves. Observations of heavy-to-extreme precipitation especially during the dissipating stages of a thunderstorm and virga (a mass of streaks of rain appearing under a cloud that evaporating before reaching ground) are strong indicators for windshear and microburst events. Pilot reports (PIREPS), surface-based low-level windshear alerting system (LLWAS) warnings at airports, and air traffic control (ATC) advisories are also reliable windshear advisories outside aircraft-generated reactive and predictive windshear detection.
The general guidance for windshear avoidance and responding to windshear alerts are not significantly different between aircraft manufacturers and aircraft types, but awareness and recommended procedures per the manufacturer should be adhered to. Windshear is most dangerous for aircraft flying at low altitudes during takeoff, approach, and landing phases of flight where the proximity to the ground may not allow for a safe recovery from a windshear episode. The presence of windshear should deter the pilot from departing and instead opting to delay takeoff until safer weather conditions prevail. Approaches to airports experiencing windshear should be discontinued and pilots should elect to either hold for favorable weather conditions or proceed to a suitable alternate airport. If windshear is suspected, the following techniques may improve the safety margins and permit recovery from a windshear encounter during takeoff, approach and landing.
During Takeoff: Pilots are encouraged to select the longest suitable runway, conduct a thorough pre-flight briefing and review key airspeeds, use maximum takeoff thrust and avoid reduced thrust takeoff settings (e.g., FLEX Temperature or Derated), maintain initial climb pitch attitude and thrust setting until a safe terrain and obstruction altitude is achieved, closely monitor and announce deviations in aircraft performance and parameters such as airspeed, vertical speed, and attitude from normal flight. Windshear at V1 or rotation speed (VR) can be particularly dangerous since there is insufficient runway distance to safely abort the takeoff. Recommendations for windshear encountered after V1 include continuing takeoff by disconnecting auto throttle (if applicable), maintaining maximum thrust, and higher pitch attitude.
During Initial Climb: Set maximum takeoff thrust, avoid turns except for obstacle clearance, closely monitor and announce deviations in aircraft performance and parameters such as airspeed, vertical speed, attitude, and Flight Path Vector (FPV) from normal flight. Avoid aircraft configuration changes (e.g., flaps and landing gear) until the aircraft has exited windshear conditions and a positive climb is achieved.
During Approach and Landing
Windshear (both horizontal, vertical, and downdrafts) may be anticipated if the onboard computed wind vector (speed and direction) up to 10nm from the runway differ substantially from the reported surface winds. To re-iterate, flight crew (especially the non-flying pilot) should closely monitor and announce deviations in aircraft performance and parameters such as airspeed, vertical speed, and attitude from a normal stabilized approach. Recommendations while commencing an approach if windshear is suspected include configuring the aircraft for landing with the minimum allowable flap setting, faster than normal approach speed, and landing on the longest suitable runway. Most importantly, avoid large thrust reductions in response to abrupt airspeed increases. If the approach becomes unstable, a go-around should be promptly executed at maximum go-around thrust and abovementioned guidance in During Initial Climb.
References
[1] Airbus, 2008: A318/A319/A320/A321 Fleet Flight Crew Training Manual.
[2] Allan, R., 2004: Making the Skies Safer. Electronic Design.
[3] Boeing, 1997: Document Number D6-27370-TBC, The Boeing Company.
[4] Bombardier, 2022: Flight Crew Reference Manual GL 6000 FCOM. Vol. 1
[5] Bowles R.L., 1990: Reducing wind shear risk through airborne systems technology. 17th Congress of the International Council of the Aeronautical Sciences, Stockholm, Sweden.
[6] Daney, J. and X. Lesceu, 2015: Wind shear: an invisible enemy to pilots? Airbus S.A.S, Safety first, #19.
[7] FAA, 1983: AC 120-41: Criteria for Operational Approval of Airborne Wind Shear Alerting and Flight Guidance.
[8] Flight Safety Foundation, 2000: FSF ALAR Briefing Note 5.4–Wind Shear. Flight Safety Digest.
[9] Lee, Y.F. and P.W. Chan, 2014: LIDAR-based F-factor for wind shear alerting: different smoothing algorithms and application to departing flights. Met. Apps, 21: 86-93.
[10] Nguyen, L., 2012: Technical standard order authorization and airworthiness approval considerations for aircraft weather radar systems. IEEE/AIAA 31st Digital Avionics Systems Conference, Williamsburg, VA, USA, 5C3-1-5C3-11.
[11] Proctor, F.H. and R.L. Bowles, 1992: Three-dimensional simulation of the Denver 11 July 1988 microburst-producing storm. Meteorol. and Atmos. Phys., 49: 107-124.